Research
We aim to understand and control the physical properties of semiconductor nanomaterials through chemical synthesis. Our approach combines material synthesis with physical measurements and in-house computational modeling to answer several general questions, such as:
- What are the fundamental limits of size, composition, and morphology that can be chemically encoded in a nanostructure?
- How does a material's morphology affect its interaction with light? Can absorption, scattering, and waveguiding characteristics be entirely controlled through nanoscale morphology?
- How do charge carriers move and recombine in semiconductor nanostructures? How can the unique characteristics of nanostructures, ranging from intense built-in fields to quantum confinement effects, be used for new solar energy devices?
The materials we develop have applications in solar and thermal energy, electronics, and photonics. By developing synthetic methods to control nanomaterial composition, morphology, and doping, we create structures that can function as (1) nanostructured solar cell devices, (2) a platform for hybrid semiconductor-molecular interfaces, or (3) one-dimensional components of photonic, electronic, and thermal-energy devices. Nanomaterial synthesis is coupled with optical and electronic experimental characterization using a range of techniques from nanofabrication to micro-spectroscopy. In-house finite-element optical and device modeling is used to interpret experimental measurements and design new materials, providing rapid feedback between synthesis, measurement, and simulation. The approach is multidisciplinary, interfacing chemistry, physics, materials science, and engineering.
Funding
UNC College of Arts and Science
http://www.college.unc.edu
Packard Foundation
http://www.packard.org/
National Science Foundation (NSF)
http://www.nsf.gov
Sloan Foundation
http://www.sloan.org
Research Corporation of Science Advancement
http://www.rescorp.org/
Department of Energy (DOE)
CHASE
http://www.solarhub.unc.edu
Nanomaterial Synthesis
CVD System and Nanowire Growth
Semiconductor nanowires are synthesized through a home-built, hot-wall chemical vapor deposition (CVD) system. This computer-controlled system enables detailed control of synthetic conditions in order to grow group IV (Si, Ge) semiconductor nanowires. Our system allows for detailed control of pressure, temperature, and flow rates of various precursor, doping, and carrier gases. This precise, automated control allows for complex coaxial and radial dopant modulation, core/shell morphologies, and even radically new nanowire geometries. For example, precise modulation of phosphorus dopants along the nanowire axis in conjunction with wet chemical etching can produce grating structures in a process termed ENGRAVE (Encoded Nanowire GRowth and Appearance through VLS and Etching).
Wet Chemical Synthesis
Our wet-chemical methods are optimized for nanoparticle synthesis. Standard equipment, including round-bottom flasks, Erlenmeyers, filter flasks, heating mantles, condensers, stir plates, and volumetric glassware, are regularly used to execute any necessary wet chemical synthesis. Our capabilities include solvothermal synthesis in addition to sol-gel techniques. A large volume multi-rotor centrifuge, Ika RV-10 rotovap with plastic coated glassware, several vacuum pumps, and vacuum oven facilitate efficient and high yield collection of prepared samples. This equipment is regularly used for synthesis of metal-oxide nanoparticles and nanoparticle pastes needed for dye-sensitized solar cell devices. Additionally, our 12 position synchronous digital magnetic stirrer with heating capabilities effectively maintains any sample requiring constant agitation. A spin coater aids in the production of homogeneous thin films. A large volume box furnace can anneal materials up to 1100 °C. Outside our own lab we have access to all the facilities and equipment available in CHANL, Chapel Hill Analytical and Nanofabrication Laboratory, and the EFRC fabrication laboratory.
Representative Publications
- "Encoding Abrupt and Uniform Dopant Profiles in Vapor-Liquid-Solid Nanowires by Suppressing the Reservoir Effect of the Liquid Catalyst" J.D. Christesen, C.W. Pinion, X. Zhang, J.R. McBride, and J.F. Cahoon. ACS Nano, 8, 11790–11798 (2014).
- "Sensitized Zinc Cobalt Oxide Spinel P-type Photoelectrodes" C.C. Mercado, A. Zakutayev, K. Zhu, C.J. Flynn, J.F. Cahoon, and A.J. Nozik. J. Phys. Chem C., 118, 25340–25349 (2014).
- "Hierarchically-Structured NiO Nanoplatelets as Mesoscale p-Type Photocathodes for Dye-Sensitized Solar Cells" C.J. Flynn, E.E. Oh, S.M. McCullough, R.W. Call, C.L. Donley, R. Lopez, J.F. Cahoon. J. Phys. Chem C., 118, 14177–14184 (2014).
- "Identifying Crystallization and Incorporation Limited Regimes During Vapor-Liquid-Solid Growth of Si Nanowires" C.W. Pinion, D.P. Nenon, J.D. Christesen, J.F. Cahoon. ACS Nano, 8, 6081-6088 (2014).
- "Synthetically Encoding 10-nm Morphology in Silicon Nanowires" J.D. Christesen,* C.W. Pinion,* E.M. Grumstrup, J.M. Papanikolas, J.F. Cahoon (*equal contribution). Nano Lett., 13, 6281-6286 (2013).
**Featured in Nature Nanotechnology Research Highlights: "Nanofabrication: Engraving Nanowires"
Nanophotonics
Plasmonics
Scattering
Absorption
The synthesis of structures with sub-wavelength dimensions gives control over light-matter interactions that are not possible with bulk materials. For example, these structures can absorb light beyond their physical cross sections (antenna effect), create localized electric field enhancements (as in SERS), and exhibit selective absorption resonances. The properties of these structures depend strongly on their morphologies. The use of synthetic techniques to control the growth of nanowires creates a wide range of optical properties among chemically similar structures. Coupling these structures to gold and other metals can induce controllable surface plasmons, further enhancing the manipulation of light.
Control of these photonic and plasmonic properties provides a path to advanced optical devices, whether to enhance the efficiency of photovoltaic cells or to develop all-optical circuit components.
ENGRAVE (Encoded Nanowire GRowth and Appearance through VLS and Etching)
Until recently, the nanowire geometry had been limited to either smooth or faceted cylinders. ENGRAVE, Encoded Nanowire Growth and Appearance through VLS and Etching, breaks these limitations and allows full control of the nanowire geometry which opens up new avenues of uses for nanowires from resistive memory to tunable SERS antennae. The geometry is controllable through the etch rate of Silicon in KOH which is dependent on the doping level of Phosphorus in Silicon. The growth and etch rates of the wire are used to calculate a dopant profile for the wire, the dopant profile is then encoded using a precisely timed computer controlled system, and finally, a timed wet chemical etch is used to reveal a complex structure. We have demonstrated this control of the ENGRAVE process to produce periodic and non-periodic gratings, sinusoids, gaps, tapers, steps, and bow-ties as seen in the figure below. Each SEM image has a red line which indicates the phosphine flow over the length pictured in the SEM images.
Representative Publications
- "Doubling Absorption in Nanowire Solar Cells with Dielectric Shell Optical Antennas" S.-K. Kim, X. Zhang, D.J Hill, K.-D. Song, J.-S. Park, H.-G. Park, and J.F. Cahoon. Nano Lett., 15, 753–758 (2015).
- "Waveguide Scattering Microscopy for Dark-Field Imaging and Spectroscopy of Photonic Nanostructures" D.J. Hill, C.W. Pinion, J.D. Christesen, J.F. Cahoon. ACS Photonics (cover article), 1, 725–731 (2014).
- "Synthetically Encoding 10-nm Morphology in Silicon Nanowires" J.D. Christesen,* C.W. Pinion,* E.M. Grumstrup, J.M. Papanikolas, J.F. Cahoon (*equal contribution). Nano Lett., 13, 6281-6286 (2013).
**Featured in Nature Nanotechnology Research Highlights: "Nanofabrication: Engraving Nanowires"
- "Horizontal silicon nanowires with radial p-n junctions: A platform for unconventional solar cells" X. Zhang,* C.W. Pinion,* J.D. Christesen, C.J. Flynn, T.A. Celano, J.F. Cahoon (*equal contribution). J. Phys. Chem. Lett. (Invited Perspective), 4, 2002-2009 (2013).
Multijunction Silicon Nanowires
Multijunction NW Growth
Nanowires are a promising platform on which to develop complex, nanostructured next-generation photovoltaic (PV) devices. For silicon nanowires, PV devices can be synthesized by encoding n-type and p-type dopants in the silicon during the wire growth. Through the VLS growth mechanism, we can encode single nanowires with multiple p-i-n junctions. The dopant transition between each successive unit is sufficiently abrupt to allow tunneling of charge carriers, and thus voltage summation across an entire wire. This allows for tunable photovoltages of up to 10 V, well in excess of what is needed to drive useful chemical reactions.
Device Fabrication
We have developed capability to synthesize prototype PV devices on length scales ranging from millimeters to hundreds of nanometers. Prototype device fabrication is enabled by shared electron-beam lithography and photolithography systems and high-quality metal evaporation capabilities in the CHANL (Chapel Hill Analytical and Nanofabrication Laboratory) user facility. Additional capabilities and equipment include printed-circuit-board (PCB) fabrication, a wire bonder, a home-built probe station, measurement system capable of femtoamp current readings, solar simulator, incident-photon-to-current-efficiency (IPCE) optical system, and scanning optical microspectroscopy.
Particle Suspension Reactors
We aim to use our multijunction silicon nanowires as particulate photocatalysts for efficienct fuel production. Our work focuses on aspects of wire design, catalyst design, and reactor engineering. We look at the water splitting reaction using solid-state co-catalysts. We have developed a method to spatioselectively deposit co-catalysts for specific reactions. We also look at using molecular catalysts with our nanowires for CO2 reduction.
Measurement and Modeling
We use finite-element modeling as a core tool to design, predict, and understand both the optical and electrical characteristics of the nanostructures, and quantitative comparison between experiment and simulation is a key scientific approach in the group. Full-field wave-optics simulations can generate accurate predictions of absorption and scattering in sub-wavelength structures. In addition, device simulations can be used to simulate drift, diffusion, and recombination processes in nanostructures, providing a detailed picture of internal fields and quantum efficiencies. Furthermore, we have used these modeling tools to predict current-voltage characteristics that can be directly compared with experiment. Finite-element simulations are performed entirely in-house using the Comsol Multiphysics commercial software. The capability to perform these simulations in house enables rapid feedback between design, synthesis, and measurement.
Representative Publications
- "Abrupt degenerately-doped silicon nanowire tunnel junctions" C. Cordoba, T. Teitsworth, M. Yang, J.F. Cahoon, K. Kavanagh. Nanotechnology, 31, 415708 (2020).
- "Interplay of Surface Recombination and Diode Geometry for the Performance of Axial p-i-n Nanowire Solar Cells" D.J. Hill, T.S. Teitsworth, E.T. Ritchie, J.M. Atkin, J.F. Cahoon. ACS Nano, 12, 10554–10563 (2018).
- "Mapping Free Carriers in Multijunction Silicon Nanowires Using Infrared Near-Field Optical Microscopy" E.T. Ritchie, D.J. Hill, T.M. Mastin, P.C. Deguzman, J.F. Cahoon, J.M. Atkin. Nano Letters, 17, 6591–6597 (2017).
Solar Fuels
Hybrid Materials for Solar Fuels
The Center for Hybrid Approaches in Solar Energy to Liquid Fuels is a DOE Energy Innovation Hub headquartered in the Chemistry Department at the University of North Carolina at Chapel Hill.CHASE includes research partners at the University of North Carolina at Chapel Hill, Yale University, Brookhaven National Laboratory, the University of Pennsylvania, North Carolina State University, and Emory University and was initiated in 2020 by the U.S. Department of Energy Office of Science, Office of Basic Energy Sciences. The CHASE mission is to develop molecule/material hybrid photoelectrodes for cooperative sunlight-driven generation of liquid fuels from carbon dioxide, nitrogen, and water. Pairing the light-absorbing properties of semiconductor materials with the selective fuel-producing reactivity of molecular catalysts, CHASE will advance a new paradigm of liquid solar fuels generation. This vast, mostly unexplored space at the intersection between molecular catalysts and heterogeneous materials presents unique opportunities for breakthroughs in photocatalyst durability and access to high-octane liquid fuels.
AI Accelerated Discovery of Solar Energy Materials
As shown by the images below, in a tandem DSPEC device light typically enters from the TiO2 photoanode, which is sensitized with a water oxidation chromophore-catalyst assembly that absorbs light in the blue to red wavelength range. The lower energy (red to infrared) light is transmitted through the water and a proton-exchange membrane to the photocathode, where light absorption with a CO2 reduction chromophore-catalyst assembly occurs. The two halves of the device, photoanode and photocathode, are connected in series and work together to efficiently generate solar fuels.
Representative Publications
- "Sensitized Zinc Cobalt Oxide Spinel P-type Photoelectrodes" C.C. Mercado, A. Zakutayev, K. Zhu, C.J. Flynn, J.F. Cahoon, and A.J. Nozik. J. Phys. Chem C., 118, 25340–25349 (2014).
- "Hierarchically-Structured NiO Nanoplatelets as Mesoscale p-Type Photocathodes for Dye-Sensitized Solar Cells" C.J. Flynn, E.E. Oh, S.M. McCullough, R.W. Call, C.L. Donley, R. Lopez, J.F. Cahoon. J. Phys. Chem C., 118, 14177–14184 (2014).
Ultrafast Dynamics
Pump-Probe Microscopy
To understand the dynamics of charge carriers in semiconductor nanostructures we probe the basic drift, diffusion, and recombination processes in single structures using ultrafast microscopy. We have developed spatially-separated pump-probe (SSPP) microscopy, a new technique which enables diffraction-limited imaging of charge carriers in space and time. Shown to the right is an SSPP time-resolved movie of charge carriers in a single silicon nanowire. At time zero, the image shows a diffraction-limited region of charge carriers. The charge carriers diffuse and recombine on a picosecond time scale, causing broadening and decay of the signal amplitude. The experiment provides direct information of the trap-state density and surface recombination velocity in semiconductor nanostructures such as silicon nanowires.
Understanding Charge Carrier Recombination
As shown by the images to the left, pump-probe microscopy provides a direct measurement of the charge carrier lifetime at a specific spatial position in a nanowire. From this lifetime, we can determine the surface recombination velocity (S) through the lifetime (τ) and diameter (d) due to the relationship, S = d/4τ. This value for S can be directly compared to our I-V simulations on photovoltaic nanowires with axial p-i-n junctions. As showing by the figures to the right, surface recombination has a dramatic effect on photovoltaic device performance. Through direct comparison of experiment and simulation, we were able to determine that the surface recombination velocity of an axial p-i-n nanowire was 7 x 103 cm/s. This value is in good agreement with the value obtained from the pump-probe measurements, S = 1.4 x 104 cm/s, for the same surface treatement. The agreement of these measurements--one from devices and simulations and the other from time-resolved microscopy--confirms the validity of both techniques and confirms that microscopy provides direct information on the physical characteristics of nanowires that determine performance in solar energy devices.
Representative Publications
- "Reversible Strain-Induced Electron-Hole Recombination in Silicon Nanowires Observed with Femtosecond Pump-Probe Microscopy" E.M. Grumstrup, M.M. Gabriel, C.W. Pinion, J.K. Parker, J.F. Cahoon, J.M. Papanikolas. Nano Lett., 14, 6287–6292 (2014).
- "Imaging Charge Separation and Carrier Recombination in Nanowire p-i-n Junctions Using Ultrafast Microscopy" M.M. Gabriel, E.M. Grumstrup, J.R. Kirschbrown, C.W. Pinion, J.D. Christesen, D.F. Zigler, E.M. Cating, J.F. Cahoon, J.M. Papanikolas. Nano Lett., 14, 3079-3087 (2014).
- "Ultrafast Carrier Dynamics in Individual Silicon Nanowires: Characterization of Diameter-Dependent Carrier Lifetime and Surface Recombination with Pump-Probe Microscopy" E.M. Grumstrup, M.M. Gabriel, E.M. Cating, C.W. Pinion, J.D. Christesen, J.R. Kirschbrown, E.L. Vallorz, J.F. Cahoon, J.M. Papanikolas. J. Phys. Chem C., 118, 8634-8640 (2014).
- "Ultrafast Carrier Dynamics of Silicon Nanowire Ensembles: The Impact of Geometrical Heterogeneity on Charge Carrier Lifetime" E.M. Grumstrup, E.M. Cating, M.M. Gabriel, C.W. Pinion, J.D. Christesen, J.R. Kirschbrown, E.L. Vallorz, J.F. Cahoon, J.M. Papanikolas. J. Phys. Chem C., 118, 8626-8633 (2014).
- "Direct Imaging of Free Carrier and Trap Carrier Motion in Silicon Nanowires by Spatially-Separated Femtosecond Pump-Probe Microscopy" M.M. Gabriel,* J.R. Kirschbrown,* J.D. Christesen, C.W. Pinion, D.F. Zigler, E.M. Grumstrup, B.P. Mehl, E. Cating, J.F. Cahoon, and J.M. Papanikolas (*equal contribution). Nano Lett., 13, 1336-1340 (2013).
**Featured in Nature Nanotechnology Research Highlights: "Charge Carriers Caught on Camera"
Electronics
Electron Ratchets
Electrical diodes for direct current and alternating current applications are typically fabricated from semiconductor p-n junctions or metal-semiconductor Schottky junctions. Each technology presents advantages and limitations in terms of the frequency of rectification, reliability of fabrication, and integration on diverse or flexible substrates. We recently reported an alternate strategy—two terminal geometric diodes—to produce zero-bias silicon diodes capable of high-frequency rectification. The geometric diodes are spatially asymmetric, two-terminal majority carrier devices operating via a ballistic mechanism, which causes the ratcheting of electrons and generation of a DC bias upon application of an AC signal. The structures are grown from the bottom-up by a vapor-liquid-solid growth process, producing single-crystalline nanowire structures with precisely controlled asymmetric geometry and doping levels. As a result, we hypothesize that with appropriate design the devices can rectify above 1 THz, and our current proof-of-concept experimental results have demonstrated rectification through at least 40 GHz. We are currently working toward understanding the design principles (synthesis conditions, surfaces, doping levels, geometry, etc) that dictate geometric diode performance and evaluating THz operation by directly coupling geometric diodes to antennas, creating rectennas that can be integrated on diverse substrates for THz detection.
Representative Publications
- "Omega-Gate Silicon Nanowire Geometric Diodes withReconfigurable Self-Switching Operation and THz Rectification", K. L. White, G. V. Rogelberg, J. P. Custer Jr., J.F. Cahoon. Advanced Electronic Materials (2023).
- "Influence of Geometry on Quasi-Ballistic Behavior in Silicon Nanowire Geometric Diodes" K. L. White, M. A. Umanstev, J. D. Low, J. P. Custer Jr., J. F. Cahoon. ACS Applied Nano Materials, 6, 5117–5126 (2023).
- "Ratcheting Quasi-ballistic Electrons in Silicon Geometric Diodes at Room Temperature" J.P. Custer, J.D. Low, D.J. Hill, T.S. Teitsworth, J.D. Christesen, C.J. McKinney, J.R. McBride, M.A. Brooke, S.C. Warren, J.F. Cahoon. Science, 368, 177-180 (2020).
**Featured in EuerkAlert! by AAAS
- "Synthetically Encoding 10-nm Morphology in Silicon Nanowires" J.D. Christesen,* C.W. Pinion,* E.M. Grumstrup, J.M. Papanikolas, J.F. Cahoon (*equal contribution). Nano Lett., 13, 6281-6286 (2013).
**Featured in Nature Nanotechnology Research Highlights: "Nanofabrication: Engraving Nanowires"
Electronics
Electron Ratchets
Electrical diodes for direct current and alternating current applications are typically fabricated from semiconductor p-n junctions or metal-semiconductor Schottky junctions. Each technology presents advantages and limitations in terms of the frequency of rectification, reliability of fabrication, and integration on diverse or flexible substrates. We recently reported an alternate strategy—two terminal geometric diodes—to produce zero-bias silicon diodes capable of high-frequency rectification. The geometric diodes are spatially asymmetric, two-terminal majority carrier devices operating via a ballistic mechanism, which causes the ratcheting of electrons and generation of a DC bias upon application of an AC signal. The structures are grown from the bottom-up by a vapor-liquid-solid growth process, producing single-crystalline nanowire structures with precisely controlled asymmetric geometry and doping levels. As a result, we hypothesize that with appropriate design the devices can rectify above 1 THz, and our current proof-of-concept experimental results have demonstrated rectification through at least 40 GHz. We are currently working toward understanding the design principles (synthesis conditions, surfaces, doping levels, geometry, etc) that dictate geometric diode performance and evaluating THz operation by directly coupling geometric diodes to antennas, creating rectennas that can be integrated on diverse substrates for THz detection.
Representative Publications
- "Omega-Gate Silicon Nanowire Geometric Diodes withReconfigurable Self-Switching Operation and THz Rectification", K. L. White, G. V. Rogelberg, J. P. Custer Jr., J.F. Cahoon. Advanced Electronic Materials (2023).
- "Influence of Geometry on Quasi-Ballistic Behavior in Silicon Nanowire Geometric Diodes" K. L. White, M. A. Umanstev, J. D. Low, J. P. Custer Jr., J. F. Cahoon. ACS Applied Nano Materials, 6, 5117–5126 (2023).
- "Ratcheting Quasi-ballistic Electrons in Silicon Geometric Diodes at Room Temperature" J.P. Custer, J.D. Low, D.J. Hill, T.S. Teitsworth, J.D. Christesen, C.J. McKinney, J.R. McBride, M.A. Brooke, S.C. Warren, J.F. Cahoon. Science, 368, 177-180 (2020).
**Featured in EuerkAlert! by AAAS
- "Synthetically Encoding 10-nm Morphology in Silicon Nanowires" J.D. Christesen,* C.W. Pinion,* E.M. Grumstrup, J.M. Papanikolas, J.F. Cahoon (*equal contribution). Nano Lett., 13, 6281-6286 (2013).
**Featured in Nature Nanotechnology Research Highlights: "Nanofabrication: Engraving Nanowires"
We aim to understand and control the physical properties of semiconductor nanomaterials through chemical synthesis. Our approach combines material synthesis with physical measurements and in-house computational modeling to answer several general questions, such as:
- What are the fundamental limits of size, composition, and morphology that can be chemically encoded in a nanostructure?
- How does a material's morphology affect its interaction with light? Can absorption, scattering, and waveguiding characteristics be entirely controlled through nanoscale morphology?
- How do charge carriers move and recombine in semiconductor nanostructures? How can the unique characteristics of nanostructures, ranging from intense built-in fields to quantum confinement effects, be used for new solar energy devices?
The materials we develop have applications in solar and thermal energy, electronics, and photonics. By developing synthetic methods to control nanomaterial composition, morphology, and doping, we create structures that can function as (1) nanostructured solar cell devices, (2) a platform for hybrid semiconductor-molecular interfaces, or (3) one-dimensional components of photonic, electronic, and thermal-energy devices. Nanomaterial synthesis is coupled with optical and electronic experimental characterization using a range of techniques from nanofabrication to micro-spectroscopy. In-house finite-element optical and device modeling is used to interpret experimental measurements and design new materials, providing rapid feedback between synthesis, measurement, and simulation. The approach is multidisciplinary, interfacing chemistry, physics, materials science, and engineering.
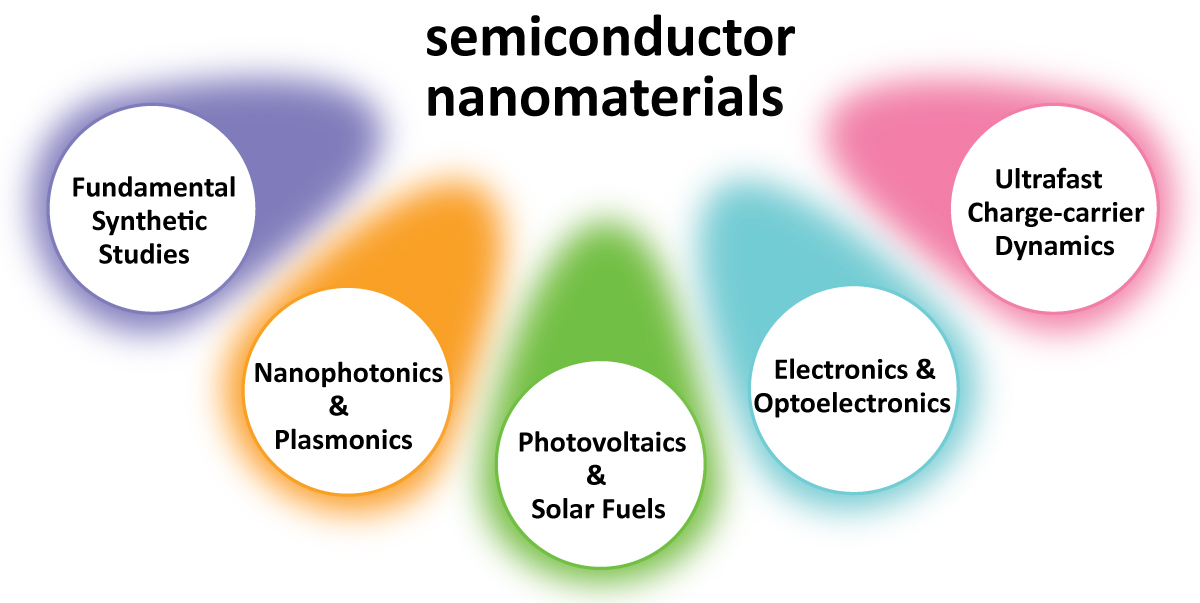
Funding
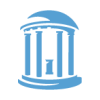
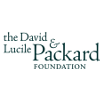
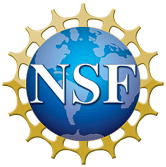
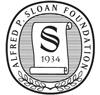
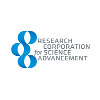
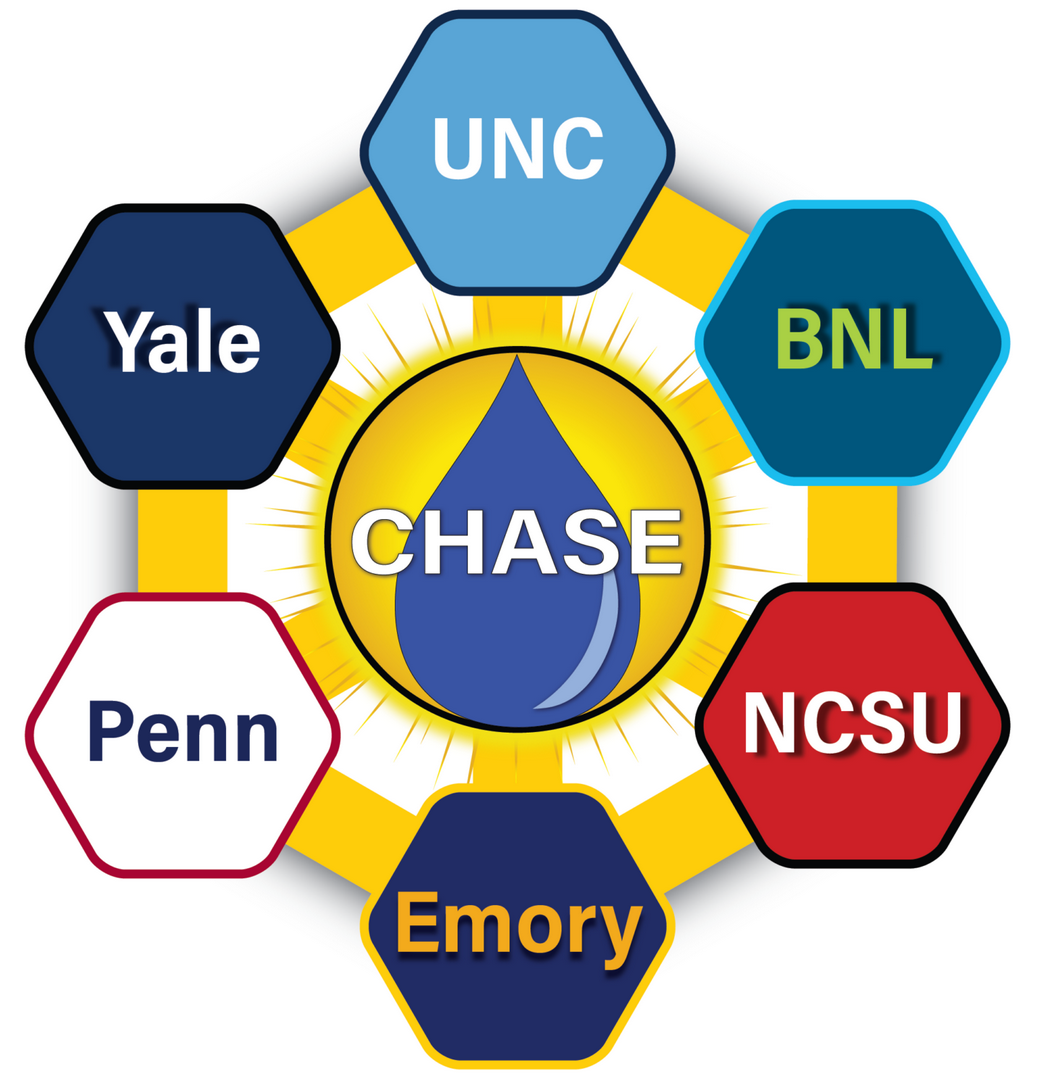
CHASE http://www.solarhub.unc.edu
Nanomaterial Synthesis
CVD System and Nanowire Growth
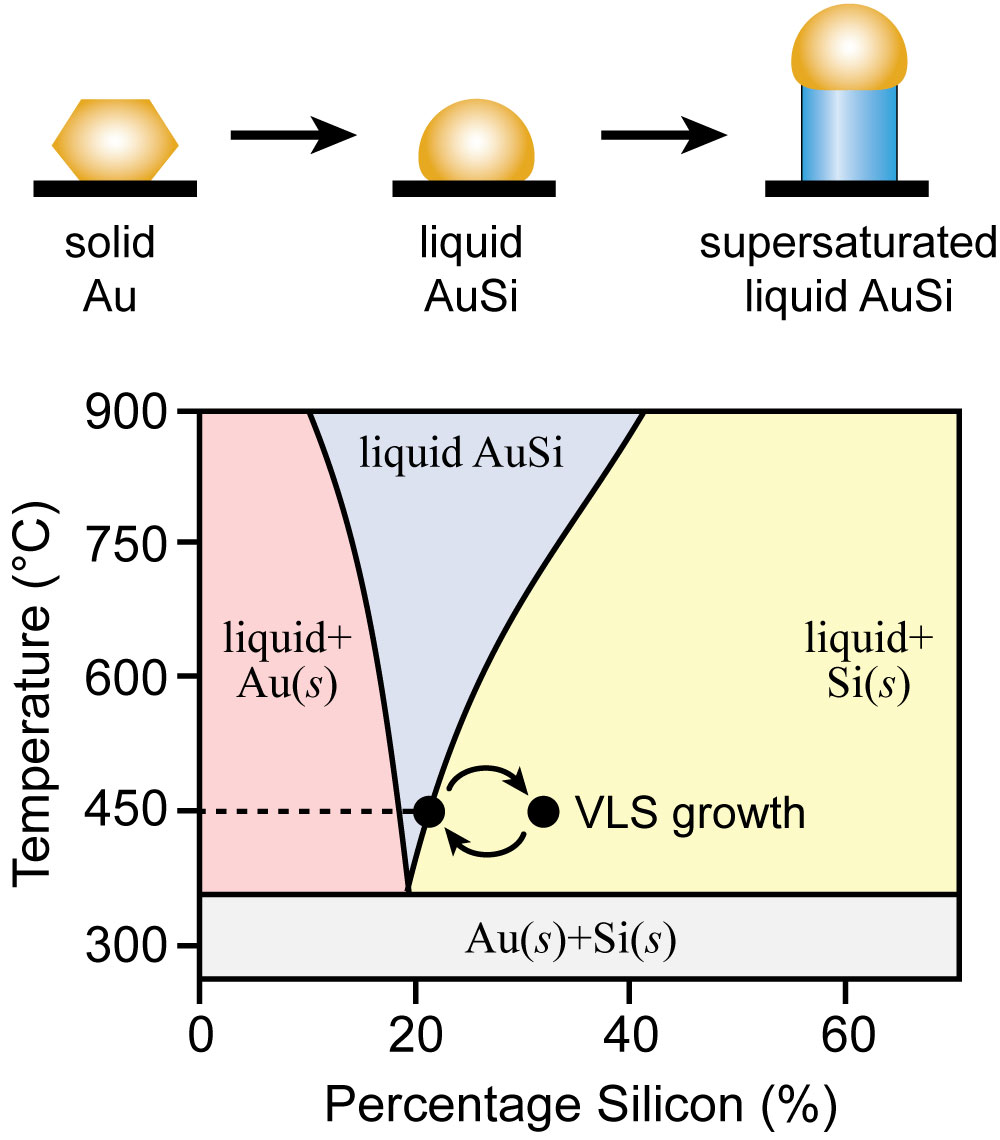
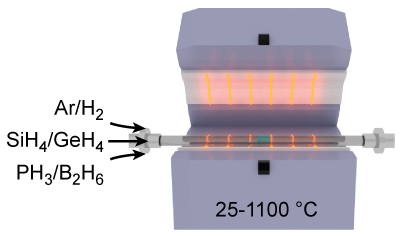
Semiconductor nanowires are synthesized through a home-built, hot-wall chemical vapor deposition (CVD) system. This computer-controlled system enables detailed control of synthetic conditions in order to grow group IV (Si, Ge) semiconductor nanowires. Our system allows for detailed control of pressure, temperature, and flow rates of various precursor, doping, and carrier gases. This precise, automated control allows for complex coaxial and radial dopant modulation, core/shell morphologies, and even radically new nanowire geometries. For example, precise modulation of phosphorus dopants along the nanowire axis in conjunction with wet chemical etching can produce grating structures in a process termed ENGRAVE (Encoded Nanowire GRowth and Appearance through VLS and Etching).

Wet Chemical Synthesis
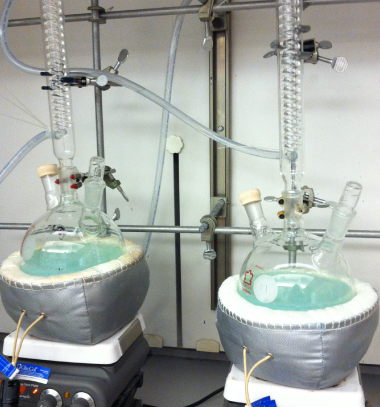
Our wet-chemical methods are optimized for nanoparticle synthesis. Standard equipment, including round-bottom flasks, Erlenmeyers, filter flasks, heating mantles, condensers, stir plates, and volumetric glassware, are regularly used to execute any necessary wet chemical synthesis. Our capabilities include solvothermal synthesis in addition to sol-gel techniques. A large volume multi-rotor centrifuge, Ika RV-10 rotovap with plastic coated glassware, several vacuum pumps, and vacuum oven facilitate efficient and high yield collection of prepared samples. This equipment is regularly used for synthesis of metal-oxide nanoparticles and nanoparticle pastes needed for dye-sensitized solar cell devices. Additionally, our 12 position synchronous digital magnetic stirrer with heating capabilities effectively maintains any sample requiring constant agitation. A spin coater aids in the production of homogeneous thin films. A large volume box furnace can anneal materials up to 1100 °C. Outside our own lab we have access to all the facilities and equipment available in CHANL, Chapel Hill Analytical and Nanofabrication Laboratory, and the EFRC fabrication laboratory.
Representative Publications
- "Encoding Abrupt and Uniform Dopant Profiles in Vapor-Liquid-Solid Nanowires by Suppressing the Reservoir Effect of the Liquid Catalyst" J.D. Christesen, C.W. Pinion, X. Zhang, J.R. McBride, and J.F. Cahoon. ACS Nano, 8, 11790–11798 (2014).
- "Sensitized Zinc Cobalt Oxide Spinel P-type Photoelectrodes" C.C. Mercado, A. Zakutayev, K. Zhu, C.J. Flynn, J.F. Cahoon, and A.J. Nozik. J. Phys. Chem C., 118, 25340–25349 (2014).
- "Hierarchically-Structured NiO Nanoplatelets as Mesoscale p-Type Photocathodes for Dye-Sensitized Solar Cells" C.J. Flynn, E.E. Oh, S.M. McCullough, R.W. Call, C.L. Donley, R. Lopez, J.F. Cahoon. J. Phys. Chem C., 118, 14177–14184 (2014).
- "Identifying Crystallization and Incorporation Limited Regimes During Vapor-Liquid-Solid Growth of Si Nanowires" C.W. Pinion, D.P. Nenon, J.D. Christesen, J.F. Cahoon. ACS Nano, 8, 6081-6088 (2014).
- "Synthetically Encoding 10-nm Morphology in Silicon Nanowires" J.D. Christesen,* C.W. Pinion,* E.M. Grumstrup, J.M. Papanikolas, J.F. Cahoon (*equal contribution). Nano Lett., 13, 6281-6286 (2013). **Featured in Nature Nanotechnology Research Highlights: "Nanofabrication: Engraving Nanowires"
Nanophotonics
Plasmonics
Scattering
Absorption
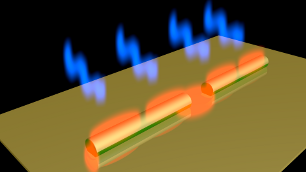
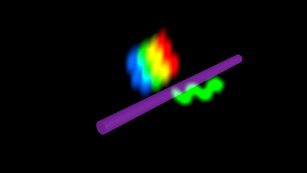
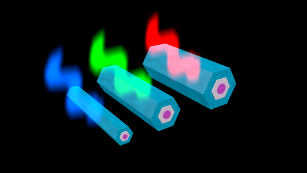
The synthesis of structures with sub-wavelength dimensions gives control over light-matter interactions that are not possible with bulk materials. For example, these structures can absorb light beyond their physical cross sections (antenna effect), create localized electric field enhancements (as in SERS), and exhibit selective absorption resonances. The properties of these structures depend strongly on their morphologies. The use of synthetic techniques to control the growth of nanowires creates a wide range of optical properties among chemically similar structures. Coupling these structures to gold and other metals can induce controllable surface plasmons, further enhancing the manipulation of light.
Control of these photonic and plasmonic properties provides a path to advanced optical devices, whether to enhance the efficiency of photovoltaic cells or to develop all-optical circuit components.
ENGRAVE (Encoded Nanowire GRowth and Appearance through VLS and Etching)
Until recently, the nanowire geometry had been limited to either smooth or faceted cylinders. ENGRAVE, Encoded Nanowire Growth and Appearance through VLS and Etching, breaks these limitations and allows full control of the nanowire geometry which opens up new avenues of uses for nanowires from resistive memory to tunable SERS antennae. The geometry is controllable through the etch rate of Silicon in KOH which is dependent on the doping level of Phosphorus in Silicon. The growth and etch rates of the wire are used to calculate a dopant profile for the wire, the dopant profile is then encoded using a precisely timed computer controlled system, and finally, a timed wet chemical etch is used to reveal a complex structure. We have demonstrated this control of the ENGRAVE process to produce periodic and non-periodic gratings, sinusoids, gaps, tapers, steps, and bow-ties as seen in the figure below. Each SEM image has a red line which indicates the phosphine flow over the length pictured in the SEM images.
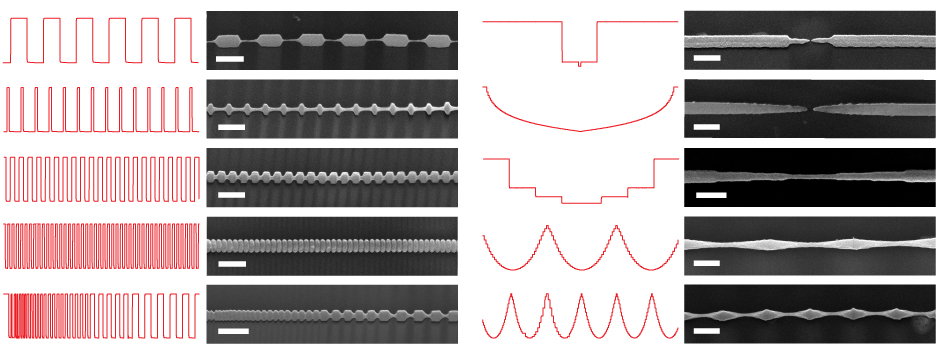
Representative Publications
- "Doubling Absorption in Nanowire Solar Cells with Dielectric Shell Optical Antennas" S.-K. Kim, X. Zhang, D.J Hill, K.-D. Song, J.-S. Park, H.-G. Park, and J.F. Cahoon. Nano Lett., 15, 753–758 (2015).
- "Waveguide Scattering Microscopy for Dark-Field Imaging and Spectroscopy of Photonic Nanostructures" D.J. Hill, C.W. Pinion, J.D. Christesen, J.F. Cahoon. ACS Photonics (cover article), 1, 725–731 (2014).
- "Synthetically Encoding 10-nm Morphology in Silicon Nanowires" J.D. Christesen,* C.W. Pinion,* E.M. Grumstrup, J.M. Papanikolas, J.F. Cahoon (*equal contribution). Nano Lett., 13, 6281-6286 (2013). **Featured in Nature Nanotechnology Research Highlights: "Nanofabrication: Engraving Nanowires"
- "Horizontal silicon nanowires with radial p-n junctions: A platform for unconventional solar cells" X. Zhang,* C.W. Pinion,* J.D. Christesen, C.J. Flynn, T.A. Celano, J.F. Cahoon (*equal contribution). J. Phys. Chem. Lett. (Invited Perspective), 4, 2002-2009 (2013).
Multijunction Silicon Nanowires
Multijunction NW Growth
Nanowires are a promising platform on which to develop complex, nanostructured next-generation photovoltaic (PV) devices. For silicon nanowires, PV devices can be synthesized by encoding n-type and p-type dopants in the silicon during the wire growth. Through the VLS growth mechanism, we can encode single nanowires with multiple p-i-n junctions. The dopant transition between each successive unit is sufficiently abrupt to allow tunneling of charge carriers, and thus voltage summation across an entire wire. This allows for tunable photovoltages of up to 10 V, well in excess of what is needed to drive useful chemical reactions.
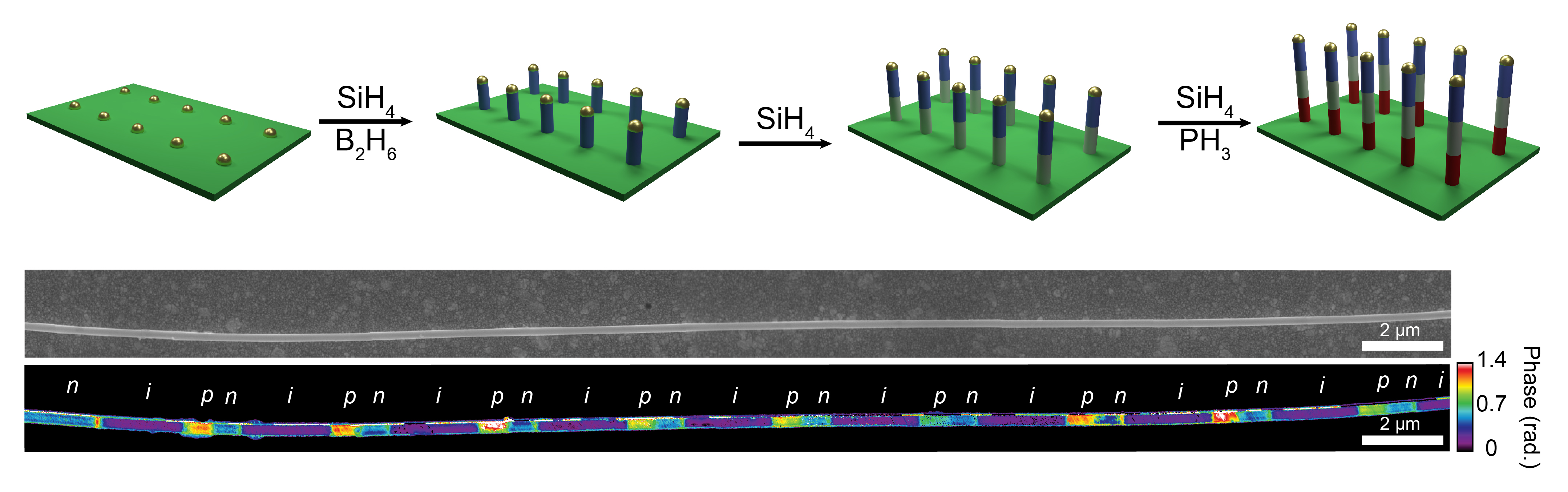
Device Fabrication
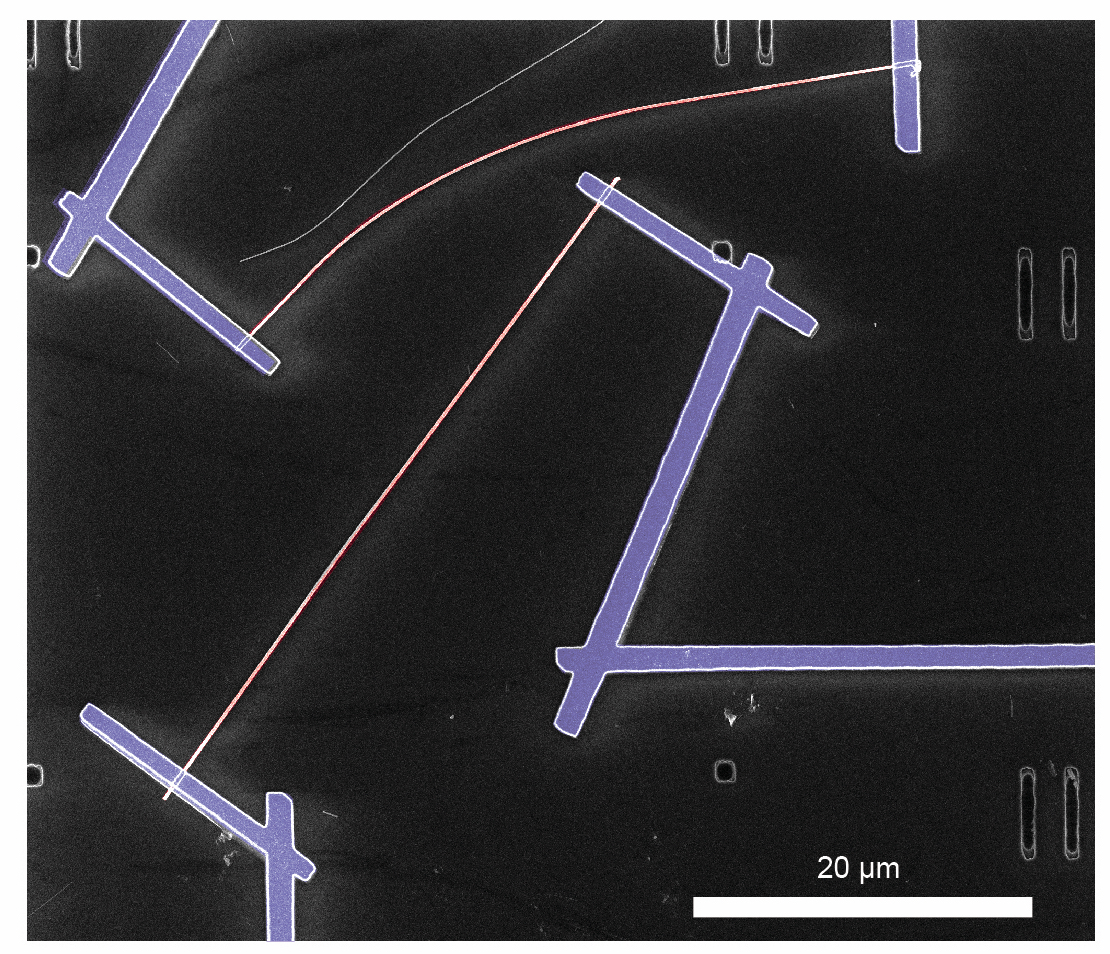
We have developed capability to synthesize prototype PV devices on length scales ranging from millimeters to hundreds of nanometers. Prototype device fabrication is enabled by shared electron-beam lithography and photolithography systems and high-quality metal evaporation capabilities in the CHANL (Chapel Hill Analytical and Nanofabrication Laboratory) user facility. Additional capabilities and equipment include printed-circuit-board (PCB) fabrication, a wire bonder, a home-built probe station, measurement system capable of femtoamp current readings, solar simulator, incident-photon-to-current-efficiency (IPCE) optical system, and scanning optical microspectroscopy.
Particle Suspension Reactors
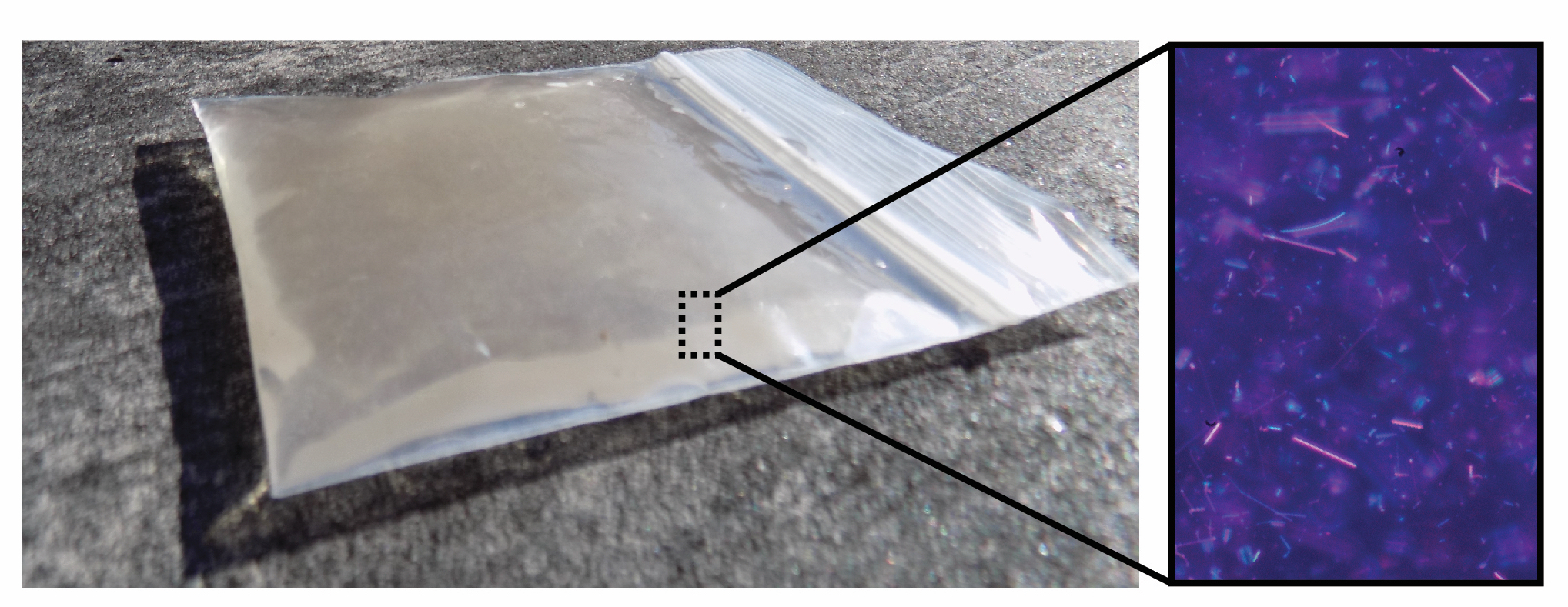
We aim to use our multijunction silicon nanowires as particulate photocatalysts for efficienct fuel production. Our work focuses on aspects of wire design, catalyst design, and reactor engineering. We look at the water splitting reaction using solid-state co-catalysts. We have developed a method to spatioselectively deposit co-catalysts for specific reactions. We also look at using molecular catalysts with our nanowires for CO2 reduction.
Measurement and Modeling
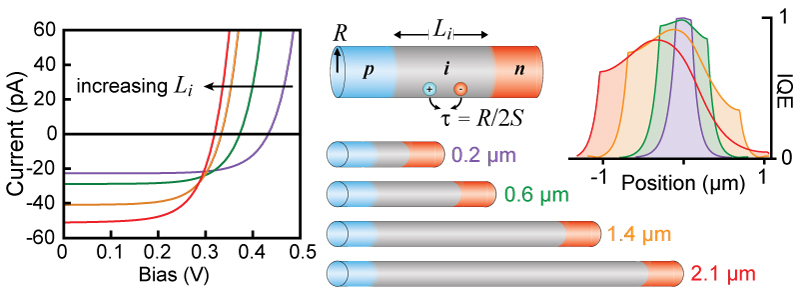
We use finite-element modeling as a core tool to design, predict, and understand both the optical and electrical characteristics of the nanostructures, and quantitative comparison between experiment and simulation is a key scientific approach in the group. Full-field wave-optics simulations can generate accurate predictions of absorption and scattering in sub-wavelength structures. In addition, device simulations can be used to simulate drift, diffusion, and recombination processes in nanostructures, providing a detailed picture of internal fields and quantum efficiencies. Furthermore, we have used these modeling tools to predict current-voltage characteristics that can be directly compared with experiment. Finite-element simulations are performed entirely in-house using the Comsol Multiphysics commercial software. The capability to perform these simulations in house enables rapid feedback between design, synthesis, and measurement.
Representative Publications
- "Abrupt degenerately-doped silicon nanowire tunnel junctions" C. Cordoba, T. Teitsworth, M. Yang, J.F. Cahoon, K. Kavanagh. Nanotechnology, 31, 415708 (2020).
- "Interplay of Surface Recombination and Diode Geometry for the Performance of Axial p-i-n Nanowire Solar Cells" D.J. Hill, T.S. Teitsworth, E.T. Ritchie, J.M. Atkin, J.F. Cahoon. ACS Nano, 12, 10554–10563 (2018).
- "Mapping Free Carriers in Multijunction Silicon Nanowires Using Infrared Near-Field Optical Microscopy" E.T. Ritchie, D.J. Hill, T.M. Mastin, P.C. Deguzman, J.F. Cahoon, J.M. Atkin. Nano Letters, 17, 6591–6597 (2017).
Solar Fuels
Hybrid Materials for Solar Fuels
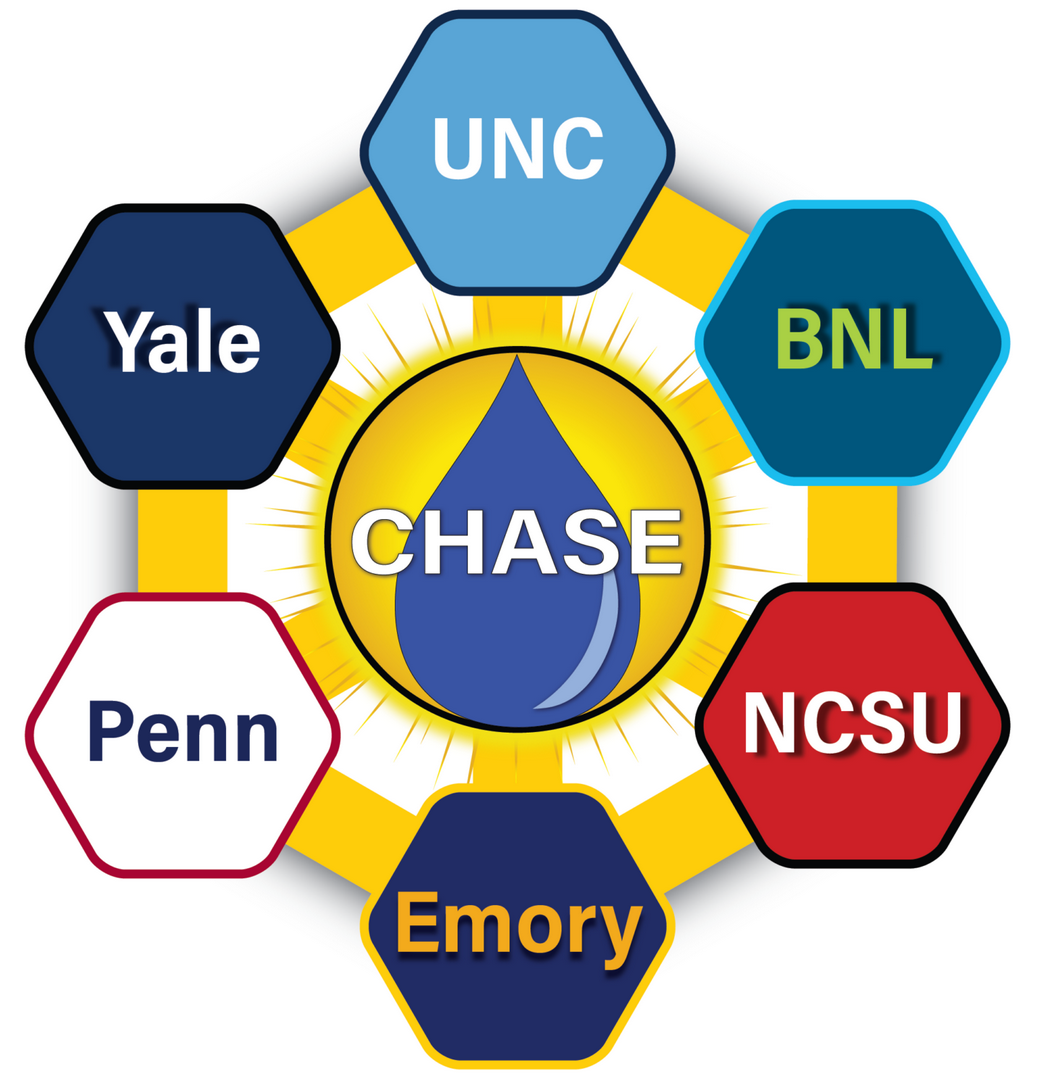
The Center for Hybrid Approaches in Solar Energy to Liquid Fuels is a DOE Energy Innovation Hub headquartered in the Chemistry Department at the University of North Carolina at Chapel Hill.CHASE includes research partners at the University of North Carolina at Chapel Hill, Yale University, Brookhaven National Laboratory, the University of Pennsylvania, North Carolina State University, and Emory University and was initiated in 2020 by the U.S. Department of Energy Office of Science, Office of Basic Energy Sciences. The CHASE mission is to develop molecule/material hybrid photoelectrodes for cooperative sunlight-driven generation of liquid fuels from carbon dioxide, nitrogen, and water. Pairing the light-absorbing properties of semiconductor materials with the selective fuel-producing reactivity of molecular catalysts, CHASE will advance a new paradigm of liquid solar fuels generation. This vast, mostly unexplored space at the intersection between molecular catalysts and heterogeneous materials presents unique opportunities for breakthroughs in photocatalyst durability and access to high-octane liquid fuels.
AI Accelerated Discovery of Solar Energy Materials
As shown by the images below, in a tandem DSPEC device light typically enters from the TiO2 photoanode, which is sensitized with a water oxidation chromophore-catalyst assembly that absorbs light in the blue to red wavelength range. The lower energy (red to infrared) light is transmitted through the water and a proton-exchange membrane to the photocathode, where light absorption with a CO2 reduction chromophore-catalyst assembly occurs. The two halves of the device, photoanode and photocathode, are connected in series and work together to efficiently generate solar fuels.
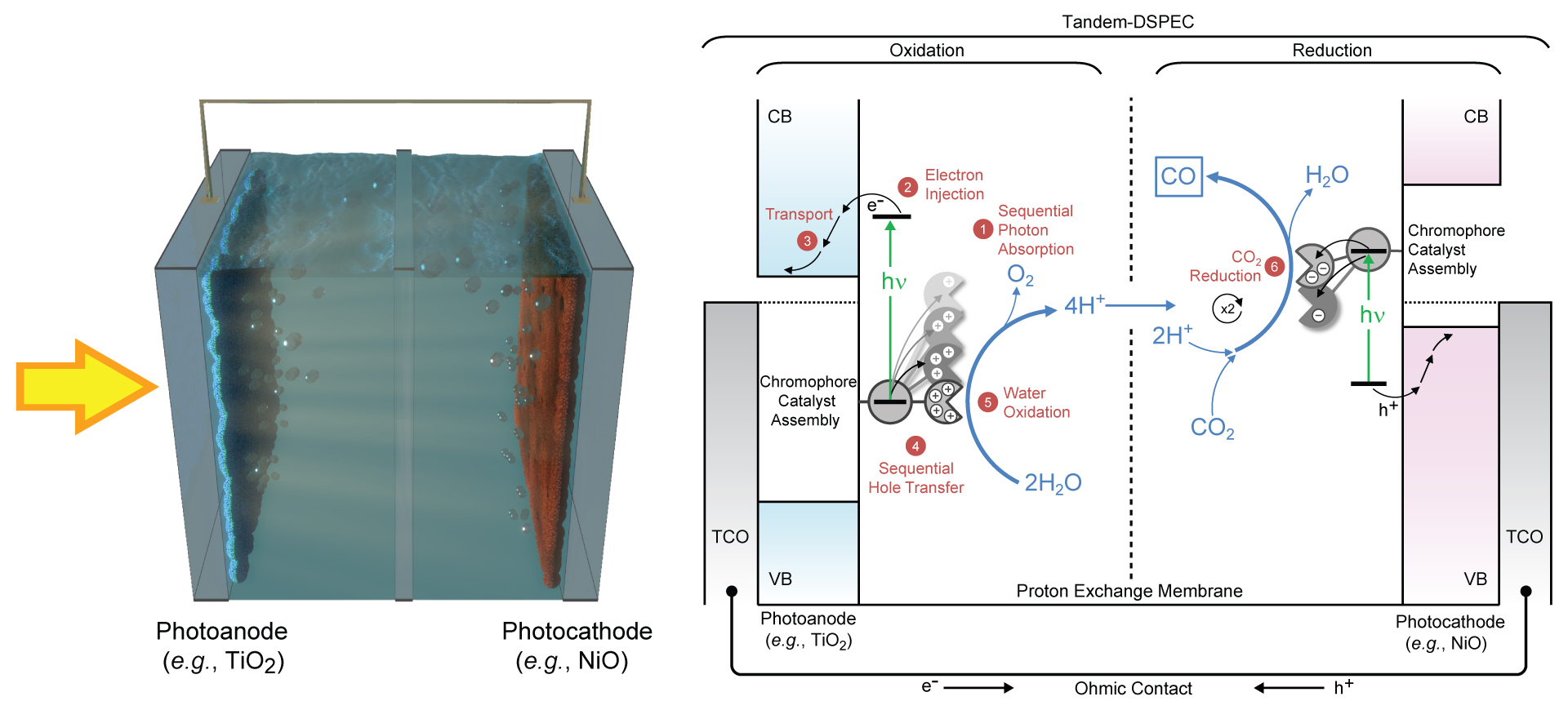
Representative Publications
- "Sensitized Zinc Cobalt Oxide Spinel P-type Photoelectrodes" C.C. Mercado, A. Zakutayev, K. Zhu, C.J. Flynn, J.F. Cahoon, and A.J. Nozik. J. Phys. Chem C., 118, 25340–25349 (2014).
- "Hierarchically-Structured NiO Nanoplatelets as Mesoscale p-Type Photocathodes for Dye-Sensitized Solar Cells" C.J. Flynn, E.E. Oh, S.M. McCullough, R.W. Call, C.L. Donley, R. Lopez, J.F. Cahoon. J. Phys. Chem C., 118, 14177–14184 (2014).
Ultrafast Dynamics
Pump-Probe Microscopy
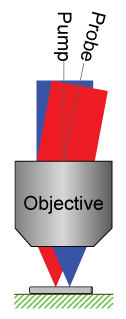
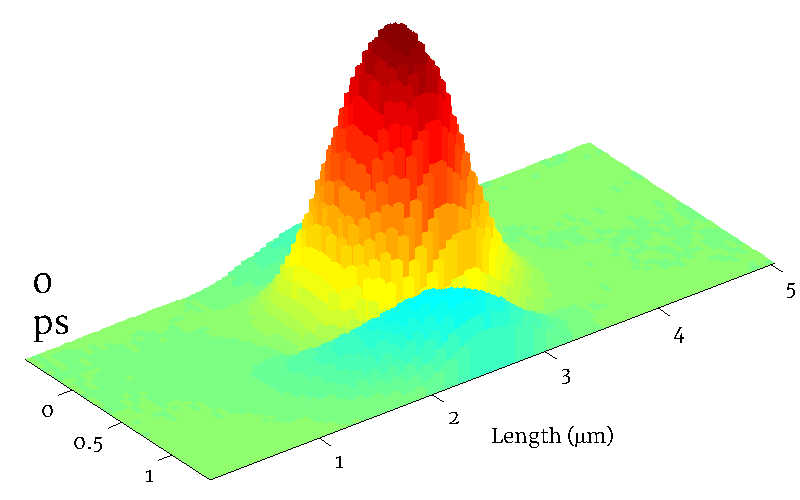
To understand the dynamics of charge carriers in semiconductor nanostructures we probe the basic drift, diffusion, and recombination processes in single structures using ultrafast microscopy. We have developed spatially-separated pump-probe (SSPP) microscopy, a new technique which enables diffraction-limited imaging of charge carriers in space and time. Shown to the right is an SSPP time-resolved movie of charge carriers in a single silicon nanowire. At time zero, the image shows a diffraction-limited region of charge carriers. The charge carriers diffuse and recombine on a picosecond time scale, causing broadening and decay of the signal amplitude. The experiment provides direct information of the trap-state density and surface recombination velocity in semiconductor nanostructures such as silicon nanowires.
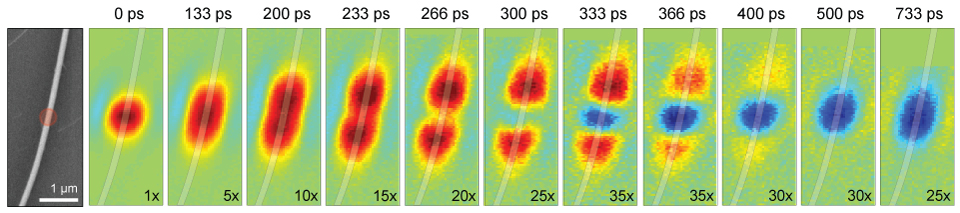
Understanding Charge Carrier Recombination
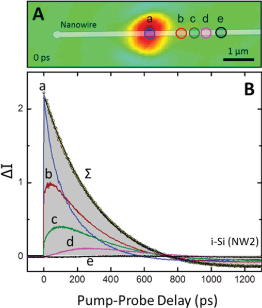
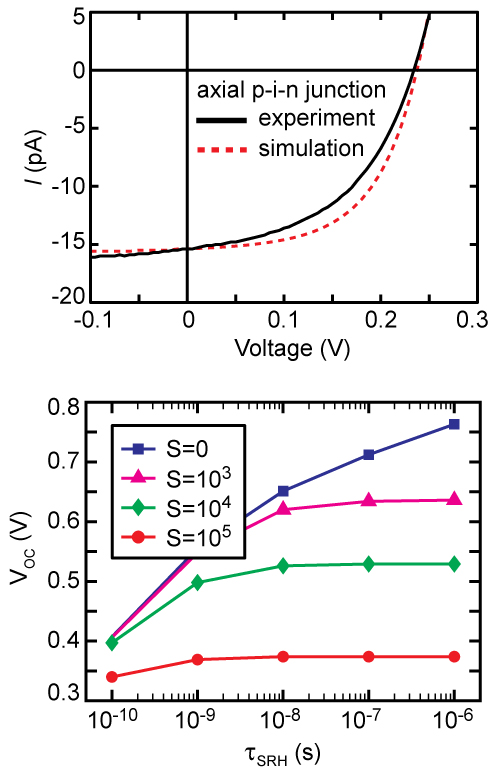
As shown by the images to the left, pump-probe microscopy provides a direct measurement of the charge carrier lifetime at a specific spatial position in a nanowire. From this lifetime, we can determine the surface recombination velocity (S) through the lifetime (τ) and diameter (d) due to the relationship, S = d/4τ. This value for S can be directly compared to our I-V simulations on photovoltaic nanowires with axial p-i-n junctions. As showing by the figures to the right, surface recombination has a dramatic effect on photovoltaic device performance. Through direct comparison of experiment and simulation, we were able to determine that the surface recombination velocity of an axial p-i-n nanowire was 7 x 103 cm/s. This value is in good agreement with the value obtained from the pump-probe measurements, S = 1.4 x 104 cm/s, for the same surface treatement. The agreement of these measurements--one from devices and simulations and the other from time-resolved microscopy--confirms the validity of both techniques and confirms that microscopy provides direct information on the physical characteristics of nanowires that determine performance in solar energy devices.
Representative Publications
- "Reversible Strain-Induced Electron-Hole Recombination in Silicon Nanowires Observed with Femtosecond Pump-Probe Microscopy" E.M. Grumstrup, M.M. Gabriel, C.W. Pinion, J.K. Parker, J.F. Cahoon, J.M. Papanikolas. Nano Lett., 14, 6287–6292 (2014).
- "Imaging Charge Separation and Carrier Recombination in Nanowire p-i-n Junctions Using Ultrafast Microscopy" M.M. Gabriel, E.M. Grumstrup, J.R. Kirschbrown, C.W. Pinion, J.D. Christesen, D.F. Zigler, E.M. Cating, J.F. Cahoon, J.M. Papanikolas. Nano Lett., 14, 3079-3087 (2014).
- "Ultrafast Carrier Dynamics in Individual Silicon Nanowires: Characterization of Diameter-Dependent Carrier Lifetime and Surface Recombination with Pump-Probe Microscopy" E.M. Grumstrup, M.M. Gabriel, E.M. Cating, C.W. Pinion, J.D. Christesen, J.R. Kirschbrown, E.L. Vallorz, J.F. Cahoon, J.M. Papanikolas. J. Phys. Chem C., 118, 8634-8640 (2014).
- "Ultrafast Carrier Dynamics of Silicon Nanowire Ensembles: The Impact of Geometrical Heterogeneity on Charge Carrier Lifetime" E.M. Grumstrup, E.M. Cating, M.M. Gabriel, C.W. Pinion, J.D. Christesen, J.R. Kirschbrown, E.L. Vallorz, J.F. Cahoon, J.M. Papanikolas. J. Phys. Chem C., 118, 8626-8633 (2014).
- "Direct Imaging of Free Carrier and Trap Carrier Motion in Silicon Nanowires by Spatially-Separated Femtosecond Pump-Probe Microscopy" M.M. Gabriel,* J.R. Kirschbrown,* J.D. Christesen, C.W. Pinion, D.F. Zigler, E.M. Grumstrup, B.P. Mehl, E. Cating, J.F. Cahoon, and J.M. Papanikolas (*equal contribution). Nano Lett., 13, 1336-1340 (2013). **Featured in Nature Nanotechnology Research Highlights: "Charge Carriers Caught on Camera"
Electronics
Electron Ratchets
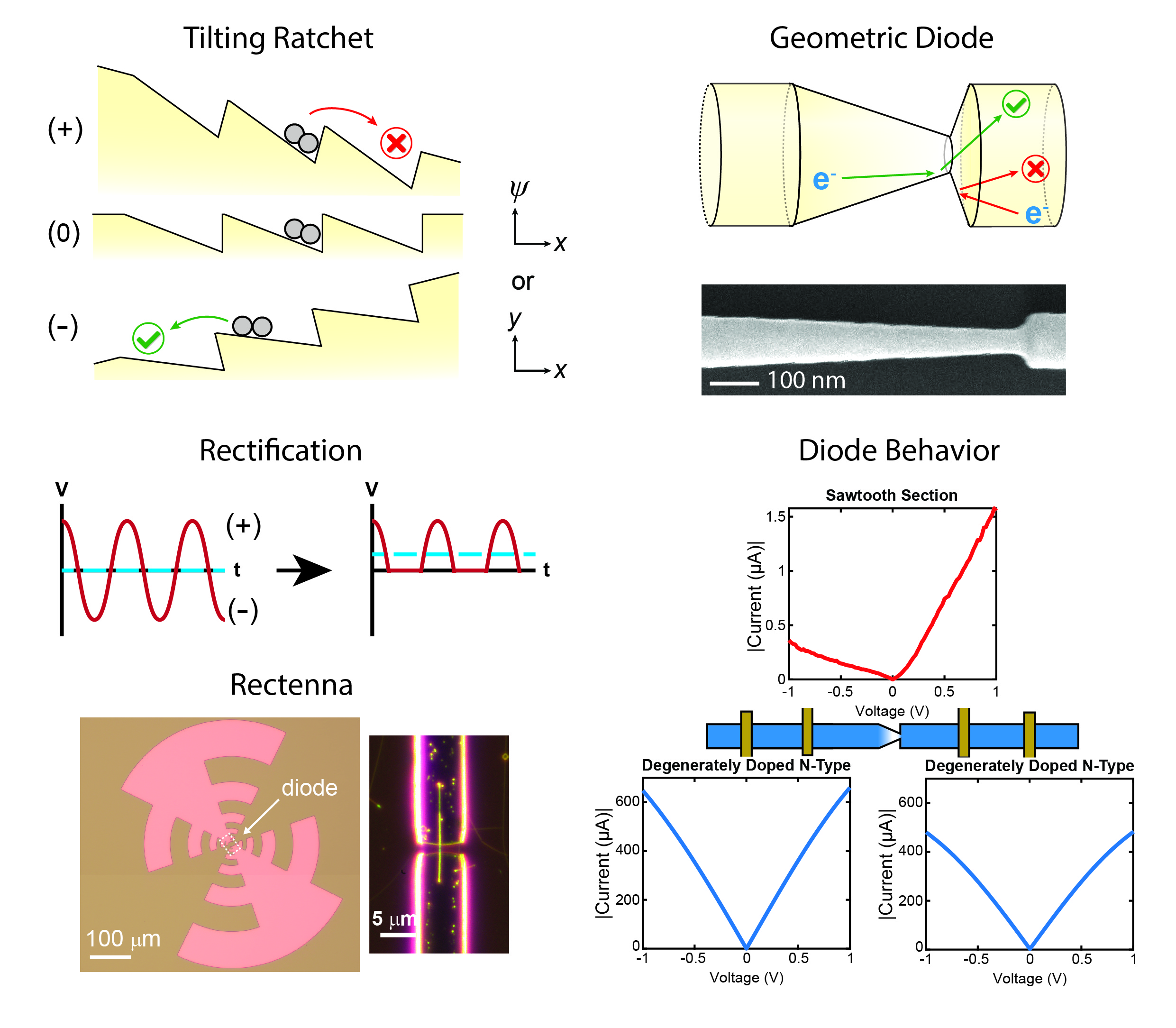
Electrical diodes for direct current and alternating current applications are typically fabricated from semiconductor p-n junctions or metal-semiconductor Schottky junctions. Each technology presents advantages and limitations in terms of the frequency of rectification, reliability of fabrication, and integration on diverse or flexible substrates. We recently reported an alternate strategy—two terminal geometric diodes—to produce zero-bias silicon diodes capable of high-frequency rectification. The geometric diodes are spatially asymmetric, two-terminal majority carrier devices operating via a ballistic mechanism, which causes the ratcheting of electrons and generation of a DC bias upon application of an AC signal. The structures are grown from the bottom-up by a vapor-liquid-solid growth process, producing single-crystalline nanowire structures with precisely controlled asymmetric geometry and doping levels. As a result, we hypothesize that with appropriate design the devices can rectify above 1 THz, and our current proof-of-concept experimental results have demonstrated rectification through at least 40 GHz. We are currently working toward understanding the design principles (synthesis conditions, surfaces, doping levels, geometry, etc) that dictate geometric diode performance and evaluating THz operation by directly coupling geometric diodes to antennas, creating rectennas that can be integrated on diverse substrates for THz detection.
Representative Publications
- "Omega-Gate Silicon Nanowire Geometric Diodes withReconfigurable Self-Switching Operation and THz Rectification", K. L. White, G. V. Rogelberg, J. P. Custer Jr., J.F. Cahoon. Advanced Electronic Materials (2023).
- "Influence of Geometry on Quasi-Ballistic Behavior in Silicon Nanowire Geometric Diodes" K. L. White, M. A. Umanstev, J. D. Low, J. P. Custer Jr., J. F. Cahoon. ACS Applied Nano Materials, 6, 5117–5126 (2023).
- "Ratcheting Quasi-ballistic Electrons in Silicon Geometric Diodes at Room Temperature" J.P. Custer, J.D. Low, D.J. Hill, T.S. Teitsworth, J.D. Christesen, C.J. McKinney, J.R. McBride, M.A. Brooke, S.C. Warren, J.F. Cahoon. Science, 368, 177-180 (2020).
**Featured in EuerkAlert! by AAAS
- "Synthetically Encoding 10-nm Morphology in Silicon Nanowires" J.D. Christesen,* C.W. Pinion,* E.M. Grumstrup, J.M. Papanikolas, J.F. Cahoon (*equal contribution). Nano Lett., 13, 6281-6286 (2013). **Featured in Nature Nanotechnology Research Highlights: "Nanofabrication: Engraving Nanowires"
Electronics
Electron Ratchets
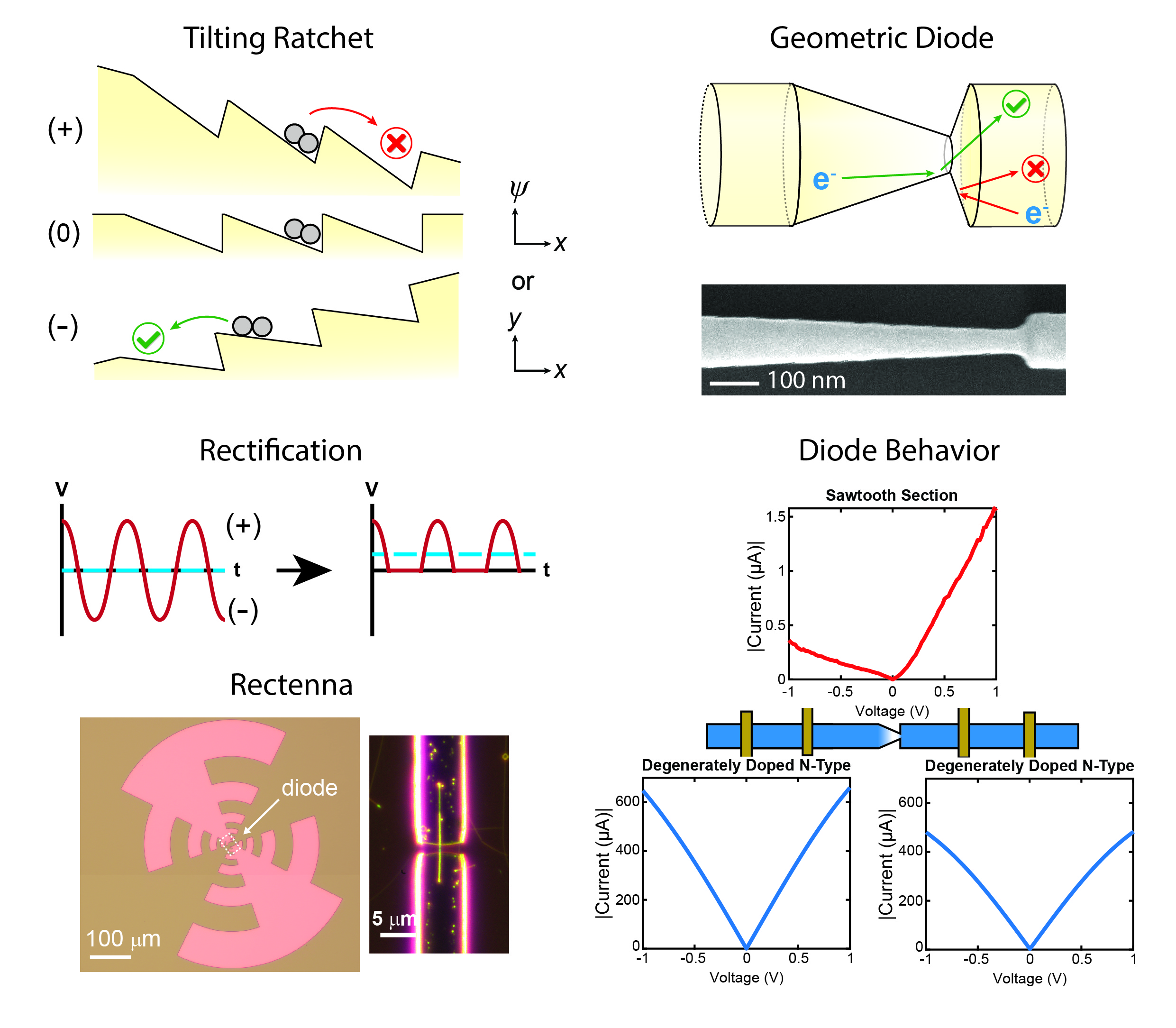
Electrical diodes for direct current and alternating current applications are typically fabricated from semiconductor p-n junctions or metal-semiconductor Schottky junctions. Each technology presents advantages and limitations in terms of the frequency of rectification, reliability of fabrication, and integration on diverse or flexible substrates. We recently reported an alternate strategy—two terminal geometric diodes—to produce zero-bias silicon diodes capable of high-frequency rectification. The geometric diodes are spatially asymmetric, two-terminal majority carrier devices operating via a ballistic mechanism, which causes the ratcheting of electrons and generation of a DC bias upon application of an AC signal. The structures are grown from the bottom-up by a vapor-liquid-solid growth process, producing single-crystalline nanowire structures with precisely controlled asymmetric geometry and doping levels. As a result, we hypothesize that with appropriate design the devices can rectify above 1 THz, and our current proof-of-concept experimental results have demonstrated rectification through at least 40 GHz. We are currently working toward understanding the design principles (synthesis conditions, surfaces, doping levels, geometry, etc) that dictate geometric diode performance and evaluating THz operation by directly coupling geometric diodes to antennas, creating rectennas that can be integrated on diverse substrates for THz detection.
Representative Publications
- "Omega-Gate Silicon Nanowire Geometric Diodes withReconfigurable Self-Switching Operation and THz Rectification", K. L. White, G. V. Rogelberg, J. P. Custer Jr., J.F. Cahoon. Advanced Electronic Materials (2023).
- "Influence of Geometry on Quasi-Ballistic Behavior in Silicon Nanowire Geometric Diodes" K. L. White, M. A. Umanstev, J. D. Low, J. P. Custer Jr., J. F. Cahoon. ACS Applied Nano Materials, 6, 5117–5126 (2023).
- "Ratcheting Quasi-ballistic Electrons in Silicon Geometric Diodes at Room Temperature" J.P. Custer, J.D. Low, D.J. Hill, T.S. Teitsworth, J.D. Christesen, C.J. McKinney, J.R. McBride, M.A. Brooke, S.C. Warren, J.F. Cahoon. Science, 368, 177-180 (2020).
**Featured in EuerkAlert! by AAAS
- "Synthetically Encoding 10-nm Morphology in Silicon Nanowires" J.D. Christesen,* C.W. Pinion,* E.M. Grumstrup, J.M. Papanikolas, J.F. Cahoon (*equal contribution). Nano Lett., 13, 6281-6286 (2013). **Featured in Nature Nanotechnology Research Highlights: "Nanofabrication: Engraving Nanowires"